The Role of Genes in Enhancing Agriculture and Horticulture
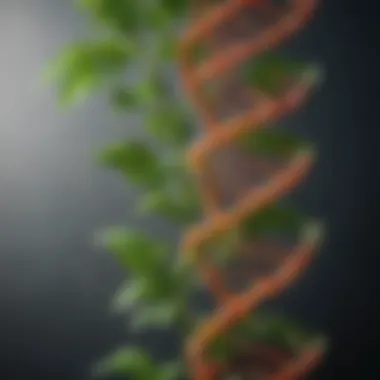
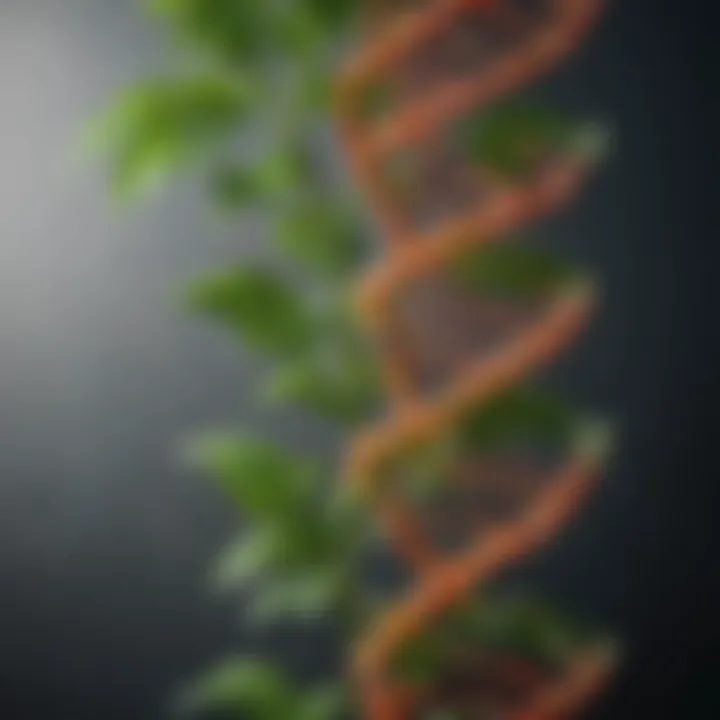
Intro
Genes serve as the foundation for the traits and characteristics we observe within all living organisms. In the context of agriculture and horticulture, they carry immense importance. A deep understanding of genetic principles allows for the enhancement of crop yield, increased disease resistance, and the promotion of more sustainable farming practices. As the global population rises, the pressure on food systems intensifies, making the study of genes more relevant than ever before.
In this exploration, we aim to uncover the profound way genes influence agricultural productivity, scrutinizing various elements from basic genetic concepts to advanced applications employed in modern practices.
Key Concepts and Terminology
Definition of Terms
To grasp the significance of genes in agriculture, it is vital to define some key terms:
- Gene: The basic unit of heredity, composed of DNA, responsible for specific traits.
- Genotype: The genetic makeup of an organism, which determines its potential characteristics.
- Phenotype: The observable characteristics of an organism, resulting from the interaction of genotype and the environment.
- Genetic Modification: The direct manipulation of an organism's genes to achieve desired traits.
Importance in Agriculture
Understanding these concepts is essential. Genes dictate crucial factors like yield quantity, taste, and resistance to environmental stresses and diseases. Selecting crop varieties based on genetic information can lead to higher productivity and better resource management.
"Genes are the blueprint of life, and agriculture hinges on manipulating that blueprint to meet our food needs more effectively."
Current Trends and Innovations
Technological Advancements
In recent years, there have been significant technological advancements in genomics that facilitate a better grasp of genetic material. Tools such as CRISPR-Cas9 enable precise editing of genes, opening new avenues for enhancing crop traits. Additionally, the use of DNA sequencing technologies streamlines the identification of beneficial genetic markers.
Sustainable Practices
Sustainability plays a vital role in modern agriculture. Genetic studies encourage the development of crops that require less water, fewer fertilizers, and reduced pesticides. Practices that emerged from genetic understanding, such as cover cropping and crop rotation, not only boost productivity but also improve soil health.
Best Practices and Techniques
Step-by-Step Guides
- Identifying Desired Traits: Farmers need to determine which traits are beneficial for their specific environments and markets before breeding or selecting crops.
- Using Genetic Tools: Engage with genetic tools like genome sequencing to identify traits. This can clarify breeding strategies and lead to more predictable outcomes.
Tools and Resources
The landscape of agricultural genetics can be explored through various resources:
- Wikipedia - An entry hub for basic genetic concepts (Wikipedia Genetics).
- Britannica - For in-depth articles on agricultural practices influenced by genetics (Britannica Genetics in Agriculture).
Maintaining a comprehensive awareness of these tools and trends enhances a farmer's ability to innovate within their practices, ensuring a more robust agricultural framework capable of supporting future food systems.
Prelude to Genes
Understanding genes is crucial to grasp the complexities of agriculture and horticulture. Genes are fundamental structures that dictate how organisms develop and function. They are tightly interwoven with every aspect of plant life. In agriculture, the management of these genetic materials is vital for improving crop performance, advancing disease resistance, and fostering sustainable practices.
This section will clarify the definition of genes, explore the structure of DNA, and discuss gene expression and regulation. Each element plays a significant role in how agricultural practices are designed and how crops are cultivated to meet modern demands. The details in this section serve as a foundation for subsequent discussions on the application of genetic knowledge in crop improvement and genetic engineering.
Definition of Genes
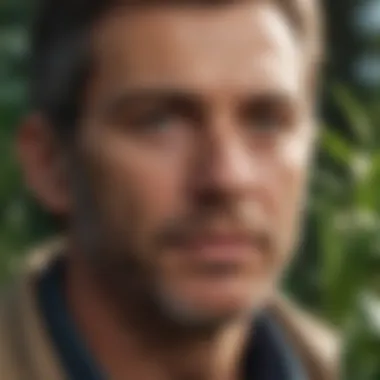
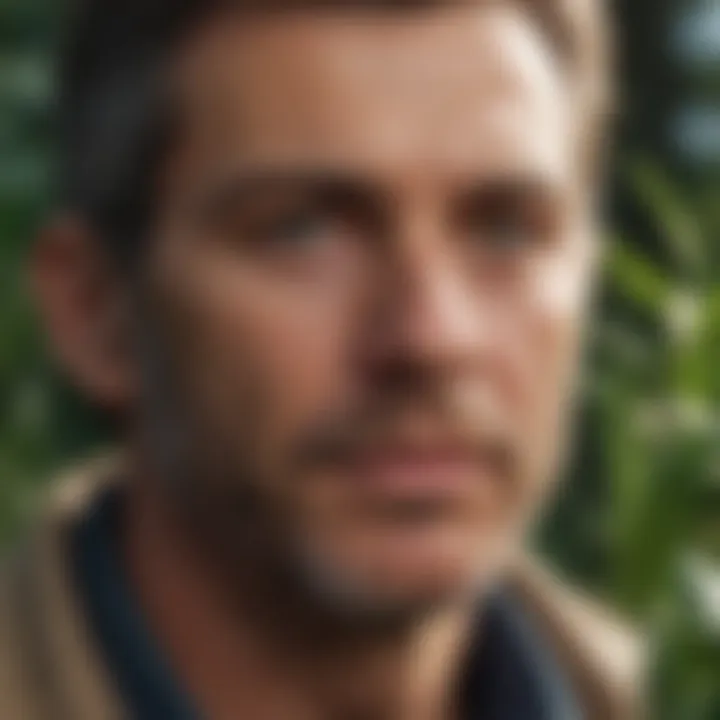
Genes can be defined as the basic units of heredity in living organisms. They are composed of sequences of DNA that encode instructions for the synthesis of proteins. Proteins are the workhorses of the cell, playing essential roles in processes such as enzymatic activity, cell structure, and signaling. Simply put, genes determine the biological attributes of an organism, influencing not only physical features but also behaviors and responses to the environment.
Basic Structure of DNA
DNA, or deoxyribonucleic acid, is the molecule that carries genetic information. Its structure consists of two strands that coil around each other, forming a double helix. Each strand is made of simpler units known as nucleotides. These nucleotides contain three components: a phosphate group, a sugar molecule, and a nitrogenous base. The sequence of these nitrogenous bases—adenine, thymine, cytosine, and guanine—forms the instructions that make up a gene. This arrangement allows DNA to be compact, yet precisely organized, ensuring accurate replication and expression within the cell.
Gene Expression and Regulation
Gene expression is the process by which the information in a gene is used to synthesize functional products, mainly proteins. This process is tightly controlled. Various factors, such as environmental conditions and developmental stages, can influence which genes are expressed at any given time. Gene regulation is fundamental for plants to adapt to changing conditions, allowing for traits like drought resistance or pest tolerance to develop as needed. Understanding these mechanisms can significantly enhance agricultural practices, leading to optimized crop yields and resilience to challenges like climate change.
"Genetic knowledge allows for better crop management and improved food security."
In summary, the exploration of genes, their structure, and their regulation sets the stage for understanding their overall impact on agriculture and horticulture. As we progress further into the article, these principles will illuminate the pathways through which genetic advancements can revolutionize farming.
The Role of Genes in Living Organisms
Genes are not merely sequences of DNA; they are the fundamental building blocks that determine the biological attributes of all living beings. Recognizing the role of genes in organisms is crucial, especially in the contexts of agriculture and horticulture. Genes serve as the instructions for producing proteins, which play roles in the development and functionality of plants and animals. This understanding empowers farmers and horticulturists to influence crop quality, disease resistance, and adaptability to changing environmental conditions.
Genetic Inheritance
Genetic inheritance is the mechanism through which traits are passed from parents to offspring. This process is a cornerstone in agriculture, guiding breeding practices and improving crop varieties. The principles of Mendelian inheritance, such as dominance and recessiveness, explain how certain traits manifest. For instance, if a farmer uses a variety of corn with a gene for high yield, their offspring may inherit this desirable trait.
The applications of genetic inheritance extend into selective breeding, where specific traits are chosen to cultivate improved plants. This traditional approach remains prevalent in agriculture, underpinning the development of resilient crop strains that thrive in local climates while yielding higher outputs.
Mutations and Variations
Mutations are changes in the DNA sequence that can lead to variations in traits among individuals. While some mutations may be harmful, others can result in beneficial characteristics, such as enhanced disease resistance or faster growth rates. In agriculture, understanding these variations can guide breeding strategies.
Farmers often seek plants with mutations that offer advantages, such as drought tolerance or increased pest resistance. It is essential to monitor these mutations, as some may lead to unintended consequences. For instance, a crop may develop resistance to a particular pest, but over time, the pest population might evolve, resulting in a need for new strategies.
Variations also contribute to the genetic diversity of crops. A genetically diverse population is more likely to adapt to environmental changes, which is a significant consideration in sustainable farming. By fostering variability within crops, farmers can ensure the long-term viability and resilience of their agricultural systems.
"Genetic variation is at the heart of agricultural innovation and sustainability."
Genes and Crop Improvement
Genes are pivotal in the realm of agriculture, directly influencing crop improvement strategies. The profound understanding of genetic principles allows farmers and scientists to develop crops that are more resilient, productive, and sustainable. At its core, crop improvement addresses the need for enhanced food security and sustainability in the face of climate change, pests, and diseases. By focusing on genetic characteristics, agricultural practices can be refined, optimizing yield and reducing resource consumption.
Selective Breeding Techniques
Selective breeding is a traditional method where plants with desirable traits are chosen to reproduce. This method has been employed for centuries. Farmers select crops based on their yield, taste, or resistance to pests. Over time, these practices have resulted in improved varieties that cater to specific environmental conditions or market demands.
The following elements highlight the significance of selective breeding:
- Traits Enhancement: By consistently selecting plants with superior characteristics, farmers can enhance traits like drought resistance or nutritional value.
- Genetic Diversity: While selective breeding can strengthen certain traits, it is important to maintain a level of genetic diversity to ensure resilience against diseases or climate variations.
- Time Efficiency: Although selective breeding can be time-consuming, its benefits are often substantial, as traits are bred into populations over generations.
Moreover, its simplicity makes it accessible to farmers without advanced technology.
Genomic Selection Approaches
Genomic selection represents a more modern approach to crop improvement, utilizing molecular markers linked to specific traits. This technique integrates advanced genomic tools to predict the performance of plants based on their genetic makeup. The implications of genomic selection are significant, providing a more precise method for breeding.
Key points regarding genomic selection include:
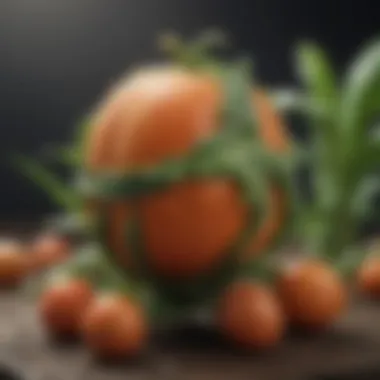
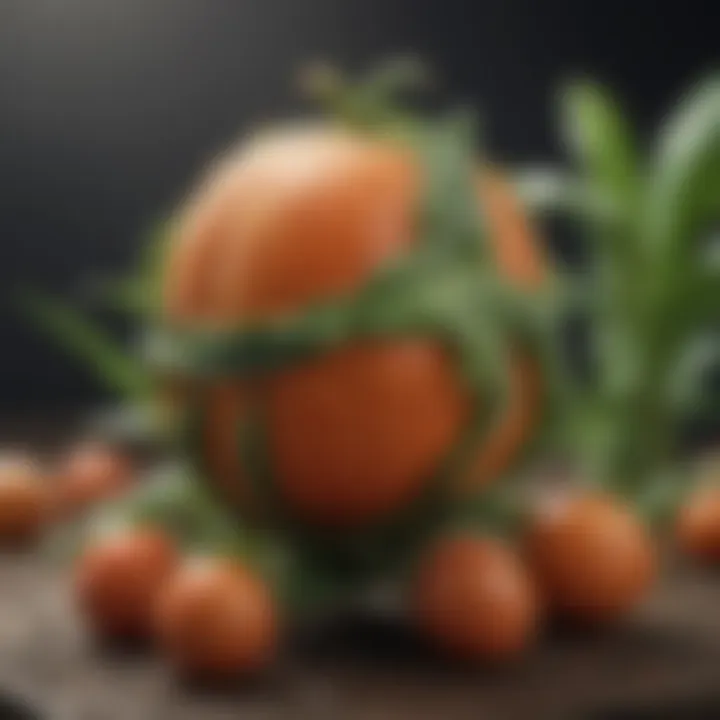
- Accuracy in Predictions: Genomic selection can enhance the accuracy of breeding programs. By analyzing genetic markers, breeders can identify plants that are more likely to produce desirable traits even before they are fully mature.
- Speed: This method accelerates the selection process, allowing for faster development of improved crop varieties, crucial for addressing urgent agricultural challenges.
- Response to Environmental Challenges: As climate conditions change, genomic selection allows researchers to quickly develop crops that can thrive under new stresses.
In summary, understanding the role of genes in crop improvement is essential. Selective breeding and genomic selection provide distinct but complementary advantages. Together, they enhance the agricultural landscape, paving the way for future developments that respond effectively to changing global demands and ensure food security.
Genetic Engineering in Agriculture
Genetic engineering represents a significant advancement in agricultural science. It allows for deliberate alterations to an organism’s genetic material. This technology enables scientists to enhance desirable traits in crops, such as yield, pest resistance, and nutritional content. Understanding genetic engineering's implications can lead farmers and horticulturists toward innovative practices that increase productivity and sustainability.
Overview of Genetic Modification
Genetic modification (GM) involves integrating specific genes into a plant’s DNA. This process may utilize techniques such as recombinant DNA technology or gene editing methods like CRISPR. By introducing new genetic material, scientists can instill traits that are not possible through traditional breeding methods. For instance, Bt corn has been engineered to express a bacterium’s protein, which is toxic to certain pests, thus reducing the need for chemical pesticides.
Benefits of GM Crops
The adoption of genetically modified crops brings numerous benefits. These include:
- Increased Yields: GM crops can show higher productivity, which is vital for feeding the growing global population.
- Pest and Disease Resistance: Naturally resistant crops decrease the risk of crop failure, which can occur due to pests or diseases.
- Reduced Chemical Usage: As seen with Bt crops, decreased reliance on pesticides can lead to lower environmental impact.
- Enhanced Nutritional Content: Certain GM crops can provide essential vitamins and minerals, addressing nutritional deficiencies.
"Genetic engineering offers a pathway to a more productive and sustainable agricultural future."
Controversies and Safety Concerns
Despite the benefits, genetic engineering also raises concerns. Public debate often centers around potential health effects and environmental impacts. Many fear that GM crops could lead to unintended consequences in ecosystems. Critics highlight:
- Potential Allergenicity: Introducing new proteins may cause allergic reactions in some individuals.
- Biodiversity Loss: The dominance of certain GM crops could threaten traditional varieties, reducing genetic diversity.
- Development of Resistant Pests: Over time, some pests may evolve to overcome genetic resistances, leading to new challenges.
Farmers and scientists must work collaboratively to navigate these challenges, ensuring that genetic engineering is applied responsibly and ethically. Understanding the balance between benefits and risks is crucial to advancing agricultural sustainability.
Impact on Sustainable Farming
The concept of sustainability in farming is becoming increasingly relevant in the context of global challenges such as climate change, population growth, and resource depletion. Understanding genes plays a pivotal role in enhancing sustainable farming practices. This section seeks to illuminate how genetic insights contribute to sustainable agriculture, laying out specific elements, benefits, and considerations that underscore its significance.
Enhancing Crop Resistance
Crop resistance to diseases and pests is vital for maintaining food security. By harnessing genetic information, farmers can develop crops that are less susceptible to leading threats. This reduces the need for chemical pesticides and fertilizers, which can have harmful effects on the ecosystem.
Breeding programs focused on gene mapping can identify traits associated with resistance. For example, genes that confer resistance to specific pathogens can be targeted through selective breeding. This not only enhances the durability of crops but also promotes better yield consistency even under unfavorable conditions.
Moreover, integrating resistant varieties into agricultural systems can create a more resilient food supply chain. As farmers grow crops that can withstand various stressors, the overall productivity improves. This strategic approach aligns with the goals of sustainable farming, as it lowers reliance on chemical inputs and mitigates environmental damage.
Reducing Environmental Impact
The environmental footprint of farming is a pressing issue, with agriculture often contributing to soil degradation, water contamination, and loss of biodiversity. Genetic advancements can lead to practices that minimize these impacts.
For instance, genetically modified organisms (GMOs) can be designed to use nutrients more efficiently, which reduces runoff into water systems. By optimizing resources, farmers can achieve significant environmental benefits.
Additionally, employing crops engineered for drought resistance helps preserve water resources. This technology allows crops to thrive with less water, contributing to conservation efforts vital in areas facing water scarcity.
"Sustainable farming methods supported by genetic advances create a win-win scenario, enhancing food security while safeguarding the planet."
In summary, recognizing the role of genes in enhancing crop resistance and reducing environmental impact paves the way for more sustainable farming practices. By leveraging genetic knowledge, the agricultural sector can advance towards a future that balances productivity with ecological integrity.
Genes in Horticulture Practices
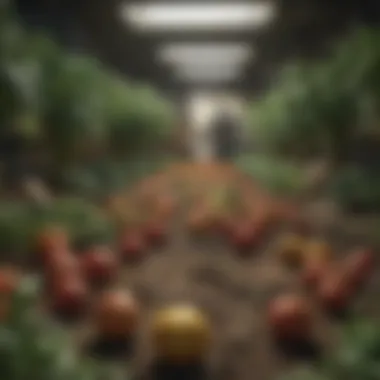
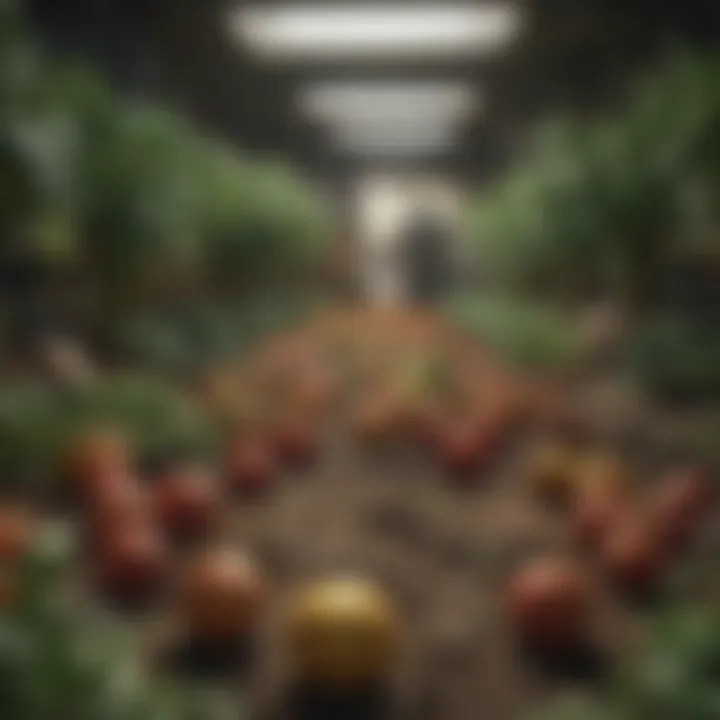
The significance of genes in horticulture practices cannot be overstated. Understanding the genetic makeup of plants enables horticulturists to make informed decisions about which varieties to cultivate. These decisions can affect not only the yields but also the quality and resilience of the crops. As farming practices evolve, the role of genetics becomes increasingly vital for ensuring that plants can adapt to changing climates, pests, and diseases.
Genetic diversity is crucial in horticulture. This diversity allows for a broader gene pool, which can be used to enhance traits such as disease resistance, growth rate, and adaptability. By cultivating a variety of genetic lines, farmers can mitigate risks associated with monoculture, such as susceptibility to pests or shifts in market demand. Moreover, maintaining genetic diversity supports ecosystem health, encouraging a balance that benefits both plants and their pollinators.
Genetic Diversity in Plants
Genetic diversity refers to the variety of genes within a particular species. In horticulture, high levels of genetic diversity are essential for sustainable crop production. It aids in increasing resilience against diseases and pests. Different genetic strains may react differently to environmental stressors, providing a buffer against crop failures.
Additionally, diverse genetics can contribute to the flavor, nutritional content, and overall market value of crops. Specific hybrid varieties may appeal to niche markets seeking unique flavors or higher nutrient densities. This adaptability is becoming increasingly important as consumer preferences shift towards more artisanal and specialty produce.
Genetic diversity is a foundation for effective farming practices and can help secure food production into the future.
Cultivation of Specialty Crops
The cultivation of specialty crops often relies heavily on understanding the genetic composition of plants. Specialty crops include herbs, spices, and ornamental plants. These crops are typically valued for their quality or unique attributes rather than bulk production. The genes influencing traits like aroma, color, and flavor are of particular interest in this sector.
To successfully cultivate specialty crops, breeders can utilize techniques such as marker-assisted selection. This method allows horticulturists to directly associate certain traits with specific genetic markers, expediting the breeding process. By selecting for these traits, farmers can elevate the quality and marketability of their crops.
In addition to market readiness, specialty crops can be crucial for biodiversity. By incorporating diverse genetic traits, chefs and consumers alike can access a range of flavors and distributions, fostering a culinary landscape that values variety.
Future Trends in Agricultural Genetics
The field of agricultural genetics is undergoing rapid changes that are set to redefine how we approach food production. Understanding these trends is crucial as they hold the potential to significantly improve farming efficiency, crop quality, and sustainability. This section explores two prominent developments in this arena: precision agriculture technologies and the CRISPR genome editing system.
Precision Agriculture Technologies
Precision agriculture encompasses a range of technologies that enable farmers to optimize their operations by using data-driven insights. This approach utilizes information gathered from various sources, such as satellite imagery, sensors, and soil analysis. The primary goal is to enhance crop yields while minimizing waste and environmental impact. Some key elements of precision agriculture include:
- Data Analytics: Advanced software interprets real-time data regarding soil health, weather patterns, and crop needs. This informs decisions on irrigation and fertilization.
- GPS Technology: Geolocation tools help in mapping fields accurately, ensuring that resources are distributed efficiently across different zones.
- Drones: Unmanned aerial vehicles can analyze crop conditions and growth patterns from above, providing insights that ground-level observations might miss.
The benefits of implementing precision agriculture go beyond simple yield increases. Farmers can reduce input costs by applying resources more efficiently. This can also decrease the carbon footprint of agricultural practices. As farmers face increasing pressure from climate change and resource constraints, adopting these technologies becomes ever more important.
"The key to feeding the future lies in meeting the challenges through innovation and technology."
CRISPR and Its Applications
Gene editing technologies, particularly CRISPR (Clustered Regularly Interspaced Short Palindromic Repeats), are revolutionizing the field of agricultural genetics. CRISPR offers a precise and efficient method for altering specific DNA sequences within an organism. This capability allows scientists to enhance traits in crops, such as disease resistance, drought tolerance, and nutritional content. Some important applications of CRISPR in agriculture include:
- Disease Resistance: By modifying genes responsible for susceptibility, crops can be made more resilient to various pathogens, reducing the need for chemical treatments.
- Drought Tolerance: Adjusting genetic pathways related to water retention can help crops survive in arid conditions, making farming viable in increasingly challenging climates.
- Enhanced Nutritional Value: CRISPR can be utilized to boost the levels of essential vitamins and minerals in crops, addressing malnutrition in vulnerable populations.
While the promise of CRISPR is significant, it also raises a few considerations. Regulatory frameworks concerning genetically modified organisms (GMOs) differ worldwide, which can affect research and development in various regions. Moreover, ethical concerns about unintended effects on ecosystems or long-term human health persist. Understanding these factors is crucial for both farmers and researchers as they navigate the future of agricultural genetics.
Each of these trends—precision agriculture and CRISPR technology—exemplifies a shift towards more scientifically informed farming practices. Together, they illuminate the path toward more sustainable and productive agricultural systems.
Culmination
Genes play an indispensable role in agriculture and horticulture. Their significance extends far beyond mere traits; they are the foundation of strategies that enhance crop yield, improve disease resistance, and promote sustainability. As we have explored throughout this article, understanding genes allows for more precise farming practices and innovative horticultural techniques.
Revisiting the Importance of Genes
To revisit, genes are crucial for several reasons. They govern not only the physical attributes of plants but also their ability to adapt to challenging environmental conditions. For instance, the genetic makeup determines a plant's resilience to drought or pests. This understanding is vital in an era where climate change poses significant threats to food security.
Moreover, the exploration of genetic diversity opens avenues for more robust agricultural systems. When farmers select crops based on genetic traits, they ensure a supply of food that is both nutritious and sustainable. Thus, genes are a linchpin in the conversation about improving global agriculture.
The Future of Agriculture and Horticulture
Looking ahead, the future of agriculture and horticulture hinges on advancements in genetic technology. Precision agriculture is becoming increasingly integral. This technique utilizes data and genetic understanding to optimize resource use, enhancing productivity while minimizing waste. As farmers adopt these technologies, both crop yield and sustainability can improve dramatically.
Additionally, innovations such as CRISPR gene editing present unprecedented opportunities. This technique allows scientists and farmers to make precise alterations in the plant genome, leading to crops that can thrive in adverse conditions. Such advances support not only food production but also biodiversity, helping to maintain healthy ecosystems.