The Impact of Plant Genes on Agricultural Innovation
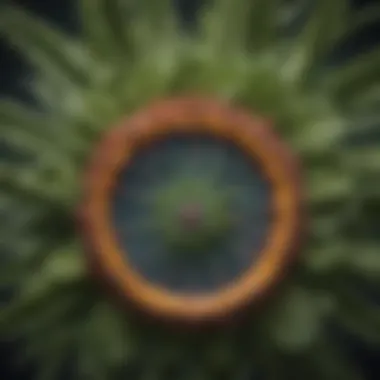
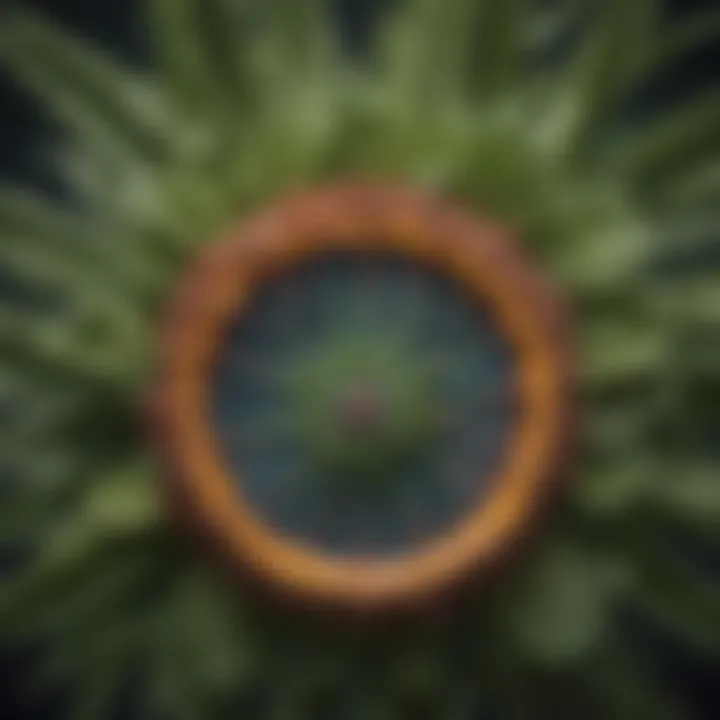
Intro
Understanding the genetic framework of plants is pivotal in enhancing agricultural practices. This area of study serves a critical role in improving not just yield but also resilience against diseases and environmental changes. The increasing global population demands innovative approaches to food production. Harnessing plant genes through advanced genetic techniques has emerged as a vital pathway for sustainable agriculture. This discussion sheds light on key terms relevant to plant genetics and examines the current trends shaping the field.
Key Concepts and Terminology
Definition of Terms
Plant genes refer to the specific sequences of DNA that determine the traits of a plant. These genes influence various aspects such as growth rate, resistance to pests, and overall productivity. Key terminology includes:
- Genetic Modification: The process of altering the genetic material of a plant to achieve desired traits. This can involve the insertion or deletion of specific genes.
- Genotype: The genetic makeup of a plant, which can significantly affect its traits and behaviors.
- Phenotype: The observable characteristics of a plant, influenced by its genotype and environment.
Importance in Agriculture
The relevance of plant genes in agriculture cannot be overstated. They are foundational to the development of new crop varieties that can withstand diseases, pests, and changing climates. Understanding these genes allows for:
- Improved crop resilience.
- Enhanced nutritional content of food products.
- More efficient agricultural practices that can lead to reduced usage of pesticides and fertilizers.
Current Trends and Innovations
Technological Advancements
Recent advancements in genetic engineering and genomic technologies are reshaping the agricultural landscape. Techniques like CRISPR-Cas9 offer precision in gene editing, enabling targeted changes that can significantly impact crop performance. Researchers are also utilizing big data analytics to understand genetic interactions better, leading to optimized breeding strategies.
Sustainable Practices
With sustainability at the forefront of agricultural discussions, the focus on plant genetics is more important than ever. Innovations in gene editing can lead to:
- Development of drought-resistant varieties, helping farmers cope with water scarcity.
- Creation of crops that require fewer chemical inputs, thus minimizing environmental impact.
"The ability to manipulate plant genetics marks a revolutionary step in agriculture, promising a future where food security and environmental health coexist."
Best Practices and Techniques
Step-by-Step Guides
Farmers interested in leveraging genetic techniques should approach their studies methodically:
- Research: Stay informed about the latest genetic advancements relevant to local crops.
- Training: Engage in workshops or courses that cover genetic principles and practices.
- Collaboration: Work with agricultural extension services or research institutions to access tools and expertise.
- Experimentation: Start small with trials of new crop varieties to assess performance and resilience.
Tools and Resources
Several resources are available to assist farmers and enthusiasts in exploring plant genetics:
- Publications: Journals such as "Plant Biotechnology Journal" publish cutting-edge research in the field.
- Online Platforms: Websites like en.wikipedia.org and britannica.com provide valuable educational content on genetics.
- Community Forums: Engaging in discussions on platforms like reddit.com can offer insights and support from fellow practitioners.
This overview lays the groundwork for a deeper exploration of plant genetics and its implications for modern agriculture. The journey through genetic research is both challenging and rewarding, paving the way for innovative agricultural solutions.
Prolusion to Plant Genetics
Understanding plant genetics is fundamental for anyone involved in agriculture. This field explores how genes influence plant traits and behaviors, creating a basis for advancements in crop production and sustainability. By delving into the structure and function of plant genes, we cultivate a deeper awareness of how genetic variations can affect productivity, disease resistance, and environmental adaptability.
The importance of plant genetics lies in its direct impact on agricultural practices. Farmers and researchers can use this knowledge to breed more resilient crops. This is particularly essential as climate change and other challenges threaten agricultural systems worldwide. Essentially, genetic insights enable the development of crops that not only yield better but also adapt to diverse environmental conditions.
Understanding Genes and Their Functions
Genes are the basic units of heredity in living organisms, acting as instructions for the development and functioning of all life forms. In plants, they determine traits like size, shape, color, and resistance to diseases. Each gene consists of a sequence of DNA, and when expressed, it directs the synthesis of proteins that manage crucial biological processes.
In plants, gene function is critical for adapting to environmental changes. For instance, certain genes might regulate responses to drought or pests. Understanding these mechanisms helps in formulating strategies to enhance trait expression beneficial for agriculture.
The Significance of Plant Genetics in Agriculture
The significance of plant genetics extends beyond academic interest. It is a practical tool that shapes modern agricultural approaches.
- Disease Resistance: Genetic studies allow scientists to identify traits that confer resistance to diseases, such as root rot or blight, which can devastate crops. By integrating these resistant genes into breeding programs, farmers can establish healthier crops.
- Yield Improvement: Through genetic analysis, researchers can understand factors that contribute to yield. This knowledge can be applied to develop high-yield varieties that are crucial for feeding a growing global population.
- Environmental Adaptability: As climate patterns shift, the ability to develop plants that can thrive in various conditions reduces dependency on chemical inputs. Breeding for traits like drought tolerance is a critical goal when considering future sustainability.
- Resource Efficiency: Utilizing plant genetics, researchers also aim to reduce the inputs required, such as water and fertilizers. This efficiency can lead to lower production costs and better environmental outcomes.
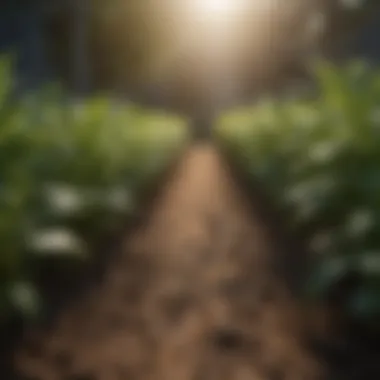
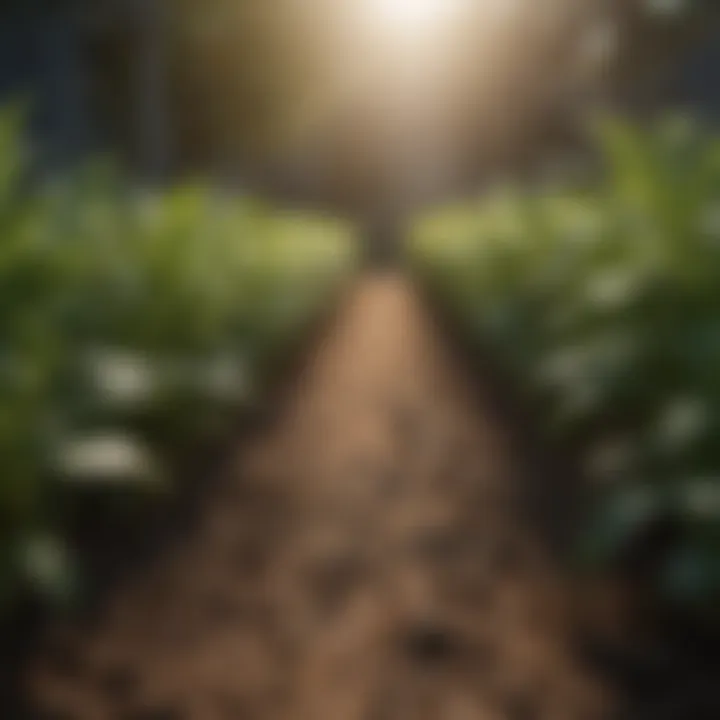
"The interplay of genetics and agriculture is vital for the future of food security and sustainable practices."
The Structure of Plant Genes
Understanding the structure of plant genes is essential to grasp how genetic information is organized, expressed, and inherited in agricultural practices. It provides insights into how traits are transferred from one generation to another, aiding in the selection and improvement of crops. Each gene contains specific sequences of DNA that dictate the synthesis of particular proteins, which in turn influence various physiological and biochemical functions in plants. By delving into the structure of plant genes, we get to see how such intricacies can lead to advancements in plant breeding and agricultural sustainability.
DNA and Chromosomes in Plants
Plants have a unique structure that governs their genetic makeup. DNA, or deoxyribonucleic acid, serves as the fundamental building block. It is organized into structures known as chromosomes. In plants, the number of chromosomes varies between species. For instance, wheat has 42 chromosomes, while Arabidopsis thaliana features only 10. Each chromosome carries a vast number of genes, and the arrangement of these genes along the chromosome is crucial for their function.
The double helix structure of DNA consists of two strands coiled around each other, forming a stable configuration. The sequence of nucleotides—adenine, thymine, cytosine, and guanine—encodes the genetic information. When researchers understand the arrangement of these bases, they can decipher the function of particular genes and anticipate how changes in one part of the DNA sequence can affect overall plant health and productivity.
"The specificity of plant genes in conjunction with their chromosomal arrangements allows for unique traits, fostering diversity within plant species and cultivars."
The study of plant chromosomes is also important for breeding programs. For example, hybridization exploits the genetic diversity found in different chromosomes, leading to enhancements such as disease resistance and increased yield. Without this foundational knowledge, improvements in plants would be significantly hampered.
Gene Expression and Regulation
Once the structure of DNA is understood, the focus shifts to gene expression and regulation. Gene expression is the process by which information from a gene is used to synthesize a functional gene product, usually a protein. This is a critical process because proteins play a role in virtually every biological function. Regulation of gene expression determines when and how much of a particular protein is made, which influences plant growth, metabolism, and responses to environmental conditions.
Regulatory elements, located near or within a gene, control transcription—this is the first step in gene expression. Transcription factors bind to these regulatory regions to either promote or inhibit gene expression. This complex interplay allows plants to adapt to various stresses, such as drought or disease, while optimizing resource use.
Moreover, advances in technology, such as RNA sequencing, allow scientists to measure gene expression levels. This technology has significantly improved the agricultural sector's ability to identify important traits and make informed decisions during plant breeding.
In summary, a deep understanding of the structure of plant genes, including DNA, chromosomes, and how they express and regulate is vital. This knowledge fundamentally shapes modern agriculture, guiding efforts to enhance crop resilience and yield through targeted genetic strategies.
Genetic Variation in Plants
Genetic variation in plants is essential for agricultural progress and sustainability. This variation refers to the diversity of genes within and among plant species. Such diversity enables plants to adapt to changing environments and resist pests and diseases. Understanding genetic variation can guide breeders to improve crop quality, yield, and resilience. Moreover, it opens the door for innovative agricultural practices, crucial for meeting global food demands. In essence, genetic variation underpins the ability of farmers to cultivate crops that thrive under diverse conditions.
Types of Genetic Variation
There are two primary types of genetic variation in plants: phenotypic variation and genotypic variation. Phenotypic variation is the observable traits resulting from the interaction of genes and the environment. Examples include differences in flower color, height, and leaf shape. On the other hand, genotypic variation refers to the genetic differences at the DNA level. These differences may not always result in observable changes but can significantly affect a plant’s characteristics, such as disease resistance or drought tolerance.
The following aspects highlight the significance of these types:
- Adaptability: A high level of phenotypic variation allows for the selection of traits better suited to specific environments.
- Breeding Opportunities: Genotypic variation is crucial for breeders in creating new varieties through hybridization.
- Population Resilience: Diverse genetic backgrounds in plant populations contribute to resilience against environmental stresses.
Sources of Genetic Variation
Genetic variation originates from multiple sources. One significant source is mutations. Mutations are random changes in the DNA sequence. They can introduce new traits into a population, influencing its genetic diversity.
Other sources include:
- Sexual Reproduction: Cross-pollination between different plants creates new gene combinations, enhancing variation.
- Gene Flow: The transfer of genes between populations through pollen dispersal contributes to genetic diversity.
- Environmental Factors: Stressors such as drought or high salinity can lead to adaptive mutations in specific plants.
"Genetic variation is at the core of our ability to produce resilient and productive crops. Without it, we would face severe challenges in agriculture and food security."
Understanding these sources provides valuable insights into how plant breeders can harness this variation for effective crop improvement. This knowledge is vital for developing strategies that enhance growth and yield while addressing the challenges posed by climate change and global food demands.
Advancements in Plant Genetics
Advancements in plant genetics have revolutionized the field of agriculture. These developments allow for the manipulation of plant genomes, leading to improved crop varieties and more efficient farming practices. The importance of these advancements cannot be overstated, as they directly address challenges such as food security and climate change. The implications of genetic research stretch far and wide, enhancing the resilience of crops against environmental changes while also increasing their nutritional value.
CRISPR and Genome Editing
CRISPR technology has gained significant attention for its ability to make precise edits in plant DNA. This method simplifies the process of altering plant genes, which was once a complex and time-consuming task. It offers a high level of specificity, allowing researchers to target particular traits, thereby improving crop resilience and productivity.
Applications in Crop Improvement
In crop improvement, CRISPR is invaluable. It allows the introduction of desired traits such as disease resistance, drought tolerance, and increased yield. One key characteristic of CRISPR is its efficiency in producing these traits without introducing foreign DNA into the plant's genome. This means that crops can be improved rapidly while maintaining their natural properties. This specificity makes CRISPR a popular choice in contemporary agricultural advancements. Some advantages include the potential for faster breeding cycles and reduced reliance on chemical pesticides. However, concerns about the unintended consequences of such genetic modifications do exist.
Ethical Considerations of CRISPR
While the applications of CRISPR are promising, the ethical considerations surrounding its use in agriculture warrant careful examination. The technology raises questions about the nature of genetic modifications and their long-term effects on ecosystems. A significant characteristic of ethical discussions on CRISPR is the debate over natural versus engineered traits. Supporters argue that these innovations can help solve pressing agricultural issues, while critics worry about biodiversity loss and potential health impacts due to untested modifications. The unique feature of this aspect lies in the balance between innovation and safety. Both benefits and drawbacks must be addressed to ensure the responsible use of CRISPR in agriculture.
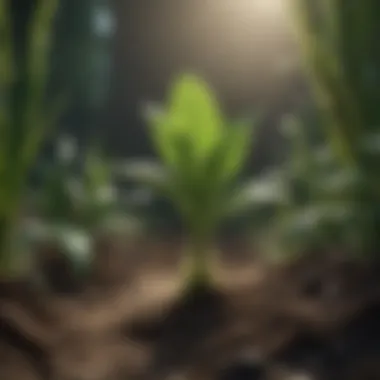
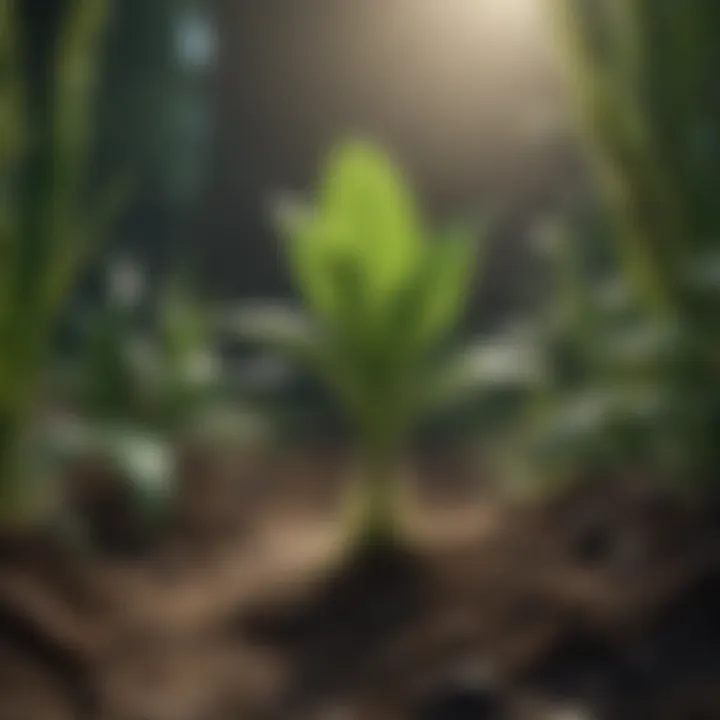
Genetic Mapping Techniques
Genetic mapping techniques play a crucial role in advancing plant genetics. These methods help scientists identify the location of genes responsible for specific traits. By understanding where these genes are situated, researchers can breed crops effective and efficiently. Key techniques include QTL (Quantitative Trait Locus) mapping and association mapping. Both methods aim to correlate specific genetic markers with traits of interest. This knowledge allows for informed decision-making in breeding programs, enhancing crop quality and resilience to pressures such as climate change. The integration of genetic mapping into agricultural practices symbolizes a step forward in harnessing plant genetics for sustainable agriculture.
Plant Breeding and Genetics
Plant breeding and genetics form the backbone of agricultural innovation. The methods used in this domain significantly influence the development of crops that can meet the growing demands of the global population. Breeding efforts focus on improving traits such as yield, disease resistance, and adaptability to varying environmental conditions. This section aims to provide an overview of the traditional and modern techniques in plant breeding, highlighting their importance and the implications they carry for future agricultural practices.
Traditional Breeding Methods
Traditional breeding methods have been the cornerstone of agricultural advancement for centuries. These methods rely on the natural genetic variation found in plants. Farmers and breeders select parent plants with desirable traits and cross them to produce offspring. This process can be time-consuming and labor-intensive but has proven effective over generations.
Key characteristics of traditional breeding include:
- Selection: Breeders choose specific plants based on observable traits such as size, taste, or resistance to disease.
- Cross-Pollination: In many crops, breeders use cross-pollination to combine traits from two different plants, leading to offspring that may inherit the best of both parents.
- Stability: Traditional methods result in stable traits but often do not produce genetically uniform crops. This variation can be beneficial for resilience in the face of pests and diseases.
While traditional methods have laid the foundation for crop improvement, they also face limitations. The time required to develop new plant varieties can span many years, which may not meet the urgent needs posed by climate change and population growth.
Modern Breeding Techniques
Modern breeding techniques leverage advances in genetic research and biotechnology. These methods enable faster and more accurate development of new plant varieties. One prominent technique is marker-assisted selection, which uses molecular markers linked to specific traits. By identifying these markers, breeders can select plants that carry desirable traits much earlier in the breeding process.
Other significant modern techniques involve:
- Genomic Selection: This approach involves predicting the breeding value of plants based on their genome. This can lead to quicker decisions in the breeding process.
- CRISPR Technology: CRISPR allows for precise editing of plant genomes. Through targeted modifications, scientists can enhance traits such as drought resistance or nutritional content.
- Tissue Culture: This technique enables the cloning of plants in a controlled environment, improving the uniformity and quality of crops.
"Understanding plant genetics is crucial for efficient breeding programs that can adapt to new challenges in agriculture."
In summary, both traditional and modern breeding techniques are essential for the future of agriculture. While traditional methods have provided a strong foundation, modern techniques offer the precision and efficiency needed to keep pace with a changing world. As researchers continue to explore the plant genome, the potential for further advances in agricultural productivity remains significant.
The Role of Plant Genes in Stress Resistance
Plant genes play a crucial role in how plants respond to various stresses, both biotic and abiotic. Understanding these genetic influences is key for developing resilient crop varieties. As agriculture faces challenges like climate change and invasive species, genes that confer stress resistance become increasingly important. These genes enable plants to survive adverse conditions, ultimately improving agricultural productivity.
Biotic Stress Resistance
Biotic stresses are caused by living organisms, including insects, fungi, bacteria, and viruses. Plant genes involved in biotic stress resistance allow plants to detect and mount defenses against these threats. For instance, genes may encode proteins that contribute to physical barriers or chemical compounds that deter pests. A notable example is the resistance genes in tomatoes, which help the plants resist bacterial wilt. These genes can trigger localized cell death to stop pathogen spread.
Moreover, the expression of these resistance genes is often mediated by signaling pathways involved in plant immunity. Understanding how to enhance these pathways can lead to more effective strategies for pest control without relying solely on chemical pesticides. This not only benefits farmers but also promotes sustainable agricultural practices.
Abiotic Stress Resistance
Abiotic stress refers to non-living environmental factors such as drought, salinity, and temperature extremes. This type of stress can significantly impact crop yields. Plant genes that contribute to abiotic stress resistance help plants tolerate unfavorable conditions. For example, the DREB (Dehydration-Responsive Element Binding) genes are involved in drought resistance. These genes play a pivotal role in enabling plants to conserve water and maintain turgor pressure under drought conditions.
Additionally, certain genes can enable plants to adapt to high salinity in soils, a rising concern in many agricultural regions caused by irrigation and climate change. With ongoing research, scientists aim to identify and manipulate these genes to breed or engineer crops that can thrive in harsh environments. This adaptive capacity improves food security in regions affected by climate variability.
"The ability of plants to resist stress is essential for sustaining high yields in fluctuating environments."
By focusing on both biotic and abiotic stress resistance, the agricultural sector can benefit from increased crop resilience. This ultimately leads to sustainable practices and enhanced productivity in ever-changing conditions. It is crucial for both researchers and practitioners to continue exploring these genetic pathways to develop innovative solutions for modern agricultural challenges.
The Connection Between Plant Genes and Yield
Understanding the relationship between plant genes and yield is essential for farmers and agricultural enthusiasts. Genes play a crucial role in determining how well plants can grow, reproduce, and withstand environmental challenges. By grasping this connection, one can improve not just crop yield but also quality and adaptability.
Genetic Factors Influencing Yield
Various genetic factors significantly influence yield. Here are some key elements to consider:
- Gene Variants: Different varieties of a crop may possess unique gene variants that affect growth patterns, fruit or grain size, and susceptibility to stress. These variations can lead to substantial differences in overall yield.
- Pathway Regulation: Specific genes regulate metabolic pathways, which are essential for photosynthesis, nutrient uptake, and energy production. Efficient pathways are critical for maximizing yield.
- Disease Resistance Genes: Plants with genes that confer disease resistance tend to thrive more than those without. This resilience allows for better yield even in challenging conditions.
- Stress Response: Genes that help plants cope with abiotic and biotic stresses can lead to improved yields. This includes responses to drought, salinity, and pest attacks.
Each of these factors reflects the intricate nature of genetic influence on yield. Understanding which genes contribute to impactful traits is a step toward making informed breeding decisions.
Case Studies of High-Yield Varieties
Several successful case studies illustrate the principles discussed above.
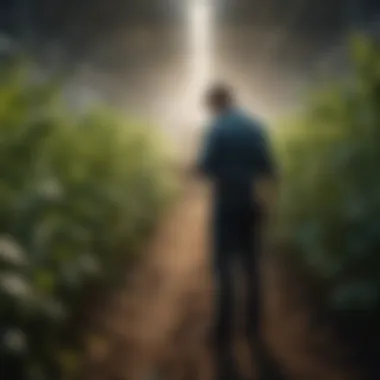
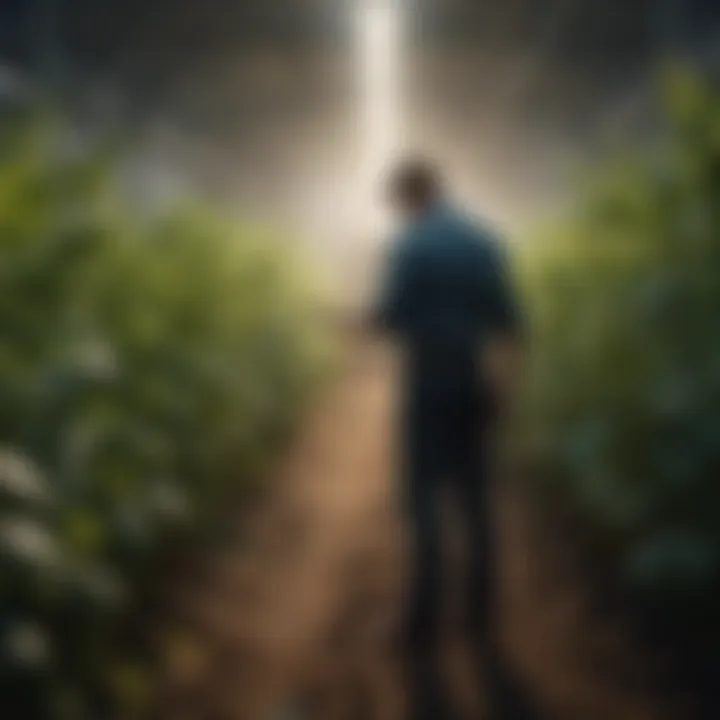
- Hybrid Maize: Hybrid varieties of maize have been bred specifically for higher yield. Crossbreeding different parent lines creates composites with enhanced traits. This practice has led to a progressive increase in maize productivity worldwide.
- IR64 Rice: Known for its high yield potential, the IR64 cultivar was created through conventional breeding techniques. It combines several favorable traits, making it popular in Asia. This variety's genes have been extensively studied for their contributions to yield and stress resilience.
- Bt Cotton: This genetically modified variety incorporates genes from the bacterium Bacillus thuringiensis to resist certain pests. Farmers growing Bt cotton often see increased yields due to reduced pest damage, showcasing the power of genetic modification.
The connection between plant genes and yield not only supports agricultural productivity but also promotes sustainable practices through enhanced resource use efficiency.
Through these examples, it becomes evident how targeted genetic research and modification can lead to varieties that yield extraordinarily well. Understanding these connections enhances the ability to make strategic decisions in crop selection and breeding, ensuring food security in an ever-changing environment.
Ethical and Economic Implications
The exploration of plant genes carries significant ethical and economic considerations that govern their application in agriculture. Understanding these implications is crucial for farmers, researchers, and policymakers alike, as they navigate the complexities of modern agricultural practices. The intersection of ethics and economics in genetic research shapes how crops are developed, marketed, and integrated into food systems. This section will analyze both ethical concerns related to genetic modification and the economic impacts of plant genetic research on the agricultural sector.
Ethics of Genetic Modification
Genetic modification in plants, while offering unprecedented advantages, brings forth a wave of ethical dilemmas. At the core, the debate centers on the rights of farmers and consumers. Concerns regarding safety often arise. Questions about the long-term effects of consuming genetically modified organisms (GMOs) persist. Regulatory frameworks struggle to keep pace with rapid advancements in genetic technology. These frameworks must ensure that GMOs are rigorously tested for safety and environmental impacts.
Moreover, the potential for genetic modification to impact biodiversity cannot be overlooked. When monoculture practices are employed, native species may become endangered. This raises questions about the moral responsibilities of agricultural producers. Farmers are now faced with decisions that weigh economic benefits against ecological risks.
Additionally, there are issues of accessibility and equity. Who benefits most from genetic advancements? Often, large agribusinesses have the resources to explore genetic technologies. Smaller farms may not have these capabilities, raising concerns about the consolidation of power within the agricultural sector.
"Ensuring that technology benefits all stakeholders requires transparency and fairness in research and distribution of resources."
Ethical considerations must thus promote inclusive practices, ensuring that the benefits of genetic research extend to a broad range of producers, not just a select few.
Economic Impact of Plant Genetic Research
The economic implications of plant genetic research are profound and multifaceted. One of the most significant benefits is increased productivity. Higher yield varieties save costs for farmers and reduce the price of food for consumers. The development of crops resistant to pests and diseases minimizes losses and reduces the need for chemical treatments, making farming more sustainable.
Investments in genetic research can lead to innovations that help address food security. As global populations rise, the demand for food will escalate. Genetic advancements allow for the cultivation of plants that can thrive in suboptimal conditions, potentially combating hunger in disadvantaged regions.
However, the economic landscape also presents challenges. The initial costs associated with genetic research and development can be prohibitive. Additionally, the market for genetically modified crops is complex, often dictated by consumer preferences and regulatory environments. Many consumers remain skeptical or opposed to GMOs. This can lead to market fluctuations, affecting profitability for farmers who choose to adopt such technologies.
Furthermore, intellectual property rights pose both opportunities and challenges. Innovations in plant genetics are often patented, which can lead to monopolistic practices. Farmers may find themselves reliant on specific seed companies, impacting their independence and profitability.
Future Directions in Plant Genetics
The exploration of plant genetics is at a pivotal junction. As the global population continues to rise, the demand for sustainable agricultural practices has never been more pressing. Future directions in plant genetics not only shape the ways we approach crop development but also ensure food security in a rapidly changing environment. Understanding these emerging trends offers critical insights into the potential of genetic research, which is essential for both current and future agricultural practices.
Innovations on the Horizon
Research in plant genetics is actively uncovering new methodologies to enhance crop resilience and yields. Notable innovations include advanced genomic technologies, such as whole-genome sequencing and automated plant phenotyping. These tools allow researchers to gain a comprehensive understanding of the genetic architecture of plants. Such insights can be applied to select traits that improve crop performance under various stresses.
Another exciting avenue is the integration of synthetic biology. This science combines engineering principles with biology to create plants that can thrive in less than ideal conditions, like drought or high salinity soils. By reengineering metabolic pathways, scientists are pioneering the development of crops that require fewer inputs yet yield greater returns.
Furthermore, precision breeding, including the use of gene editing tools like CRISPR, enhances the ability to make targeted changes in plant genomes. These innovations present vast potential for environmental sustainability, allowing for the creation of crops that reduce the need for chemical fertilizers or pesticides. In the vast field of plant genetics, innovations are continually helping push the boundaries of what is possible in agriculture.
"The next generation of farmers will rely heavily on data-driven agricultural methods to enhance yields and minimize environmental footprints."
The Importance of Continued Research
Continuing research in plant genetics is paramount for several reasons. First, ongoing studies help us understand plant responses to climate change. As the environment shifts, crops must adapt. For instance, identifying genes that confer drought resistance is critical as water scarcity becomes more common.
Second, research fosters the improvement of disease and pest resistance, which is essential for sustainable farming. Developing varieties that can withstand certain pathogens or invasive insect populations can significantly reduce dependence on chemical pesticides, offering ecological benefits.
Moreover, collaboration across disciplines—from genomics to agronomy—enhances the pace of breakthroughs. Engaging farmer communities in research fosters practical applications directly relevant to their needs. As farmers become increasingly involved in the research process, they can provide invaluable feedback, making the transition from lab to field smoother.
In summary, the future of plant genetics looks promising. Innovations on the horizon coupled with continuous research efforts hold the key to addressing some of agriculture's most pressing challenges. Plant genetics research will secure a more sustainable, resilient food system while ensuring a brighter future for both farmers and consumers.
Culmination
The conclusion of this article synthesizes the essential discussions surrounding the topic of plant genetics and its profound influence on agriculture. Understanding the roles of plant genes is not merely an academic exercise but a necessity in achieving sustainable agricultural practices. The nuances presented throughout the sections highlight key benefits derived from genetic studies, such as enhanced crop yield, resilience against diseases and environmental stress, and the promotion of biodiversity. These factors are critical in the context of global food security and the increasing challenges posed by climate change.
Summarizing the Importance of Plant Genes
Plant genes form the foundation of agricultural innovation. Their study reveals how genetic variations can improve crucial traits like nutrient uptake, drought tolerance, and pest resistance. Such insights not only facilitate the creation of high-performing plant varieties but also ensure these varieties can adapt to changing climate conditions. Understanding plant genetics helps farmers select the right crops, increasing productivity and reducing reliance on chemical treatments, which can be harmful to ecosystems.
The ongoing research in plant genetics underscores the potential for breakthroughs that could revolutionize agriculture. For instance, by unlocking the genetic codes of essential crops like rice and maize, scientists can engineer varieties that are more nutritious, require fewer resources, and yield more under adverse conditions. The importance of this cannot be overstated, as it directly impacts food supply chains and livelihoods across the world.
Encouraging Sustainable Practices in Agriculture
As discussions on sustainability grow, the role of plant genetics in promoting environmentally friendly practices becomes increasingly significant. By harnessing genetic research, agricultural systems can be made more productive without depleting natural resources. This approach aligns with the principles of sustainable agriculture, which advocates for methods that protect the environment while satisfying the food demands of a growing population.
Farmers are encouraged to adopt genetic advancements that contribute to sustainability. Examples include varieties that require less water or are resistant to pests, allowing for reduced pesticide use. This shift not only lowers production costs but also minimizes environmental impact. Through education and access to genetic resources, farmers can be empowered to implement these practices, ensuring the long-term health of both their farms and the planet.
"The study of plant genes is not just a technical endeavor; it is a pathway to ensure our agricultural systems are resilient and sustainable in the face of future challenges."